White Paper
New Opportunities in Fusion Power
Executive Summary
Solving the climate crisis will require the world to move rapidly from greenhouse gas-emitting fossil-fuel energy to abundant clean energy. That transition will be dramatically hastened if the clean energy alternatives are much cheaper than the fossil fuel sources.
Over the past fifteen years, there has been extraordinary progress with respect to intermittent renewable energy sources, specifically, solar and wind power. In favorable environments, they are now cheaper than fossil fuels when the sun is shining and the wind is blowing. Yet, today’s technologies, though, face certain fundamental limitations: solar and wind are variable energy sources, batteries are less energy-dense than liquid fuels, and biofuels compete with food.
The world also needs power sources that are firm (non-intermittent), on-demand (can be ramped up and down), ubiquitous (siteable anywhere), inexpensive (much cheaper than fossil fuels), resource-light, and safe.
Fusion is, in principle, an obvious answer. Fusion is the process that powers the stars, in which light atoms (like hydrogen) are squeezed together to form heavier atoms (like helium), thereby releasing massive amounts of energy with zero CO2 emissions. It fulfills the criteria above. And, thanks to the astronomical energy density of fusion reactions (millions of times higher energy per unit mass than in chemical reactions), fusion fuels are effectively limitless and free, with millions/billions of years of easily-accessible energy reserves.
The idea of fusion power is old, having been proposed more than 70 years ago. Moreover, the basic physics is well understood: a particular fuel (eg., deuterium-tritium, or D-T) confined at a pressure (P) and temperature (T) for a certain time (τ) can achieve sufficient fusion rates to release net-positive power provided that the product Pτ and the temperature T exceed certain critical thresholds. (Figure A shows the required thresholds for various values of P and τ.)
The problem has been that converting the theory into practical commercial fusion power has remained just over the horizon.
Fig A. A D-T fusion system must achieve a sufficiently high value of the Lawson product Pτ (at a temperature of at least ~10 keV) to release net-positive power. Scientific breakeven (power in charged fusion products exceeding plasma heating power) requires Pτ ~10 bar-s (dotted angled line), and practical systems require Pτ ~ 30-100 bar-s to achieve system-level net-positive power. Concepts operating at P > 10-2 Mbar must be pulsed, and concepts that achieve P > 105 Mbar can ignite (allowing a fusion burn to propagate into cold fuel, significantly increasing attainable yield).
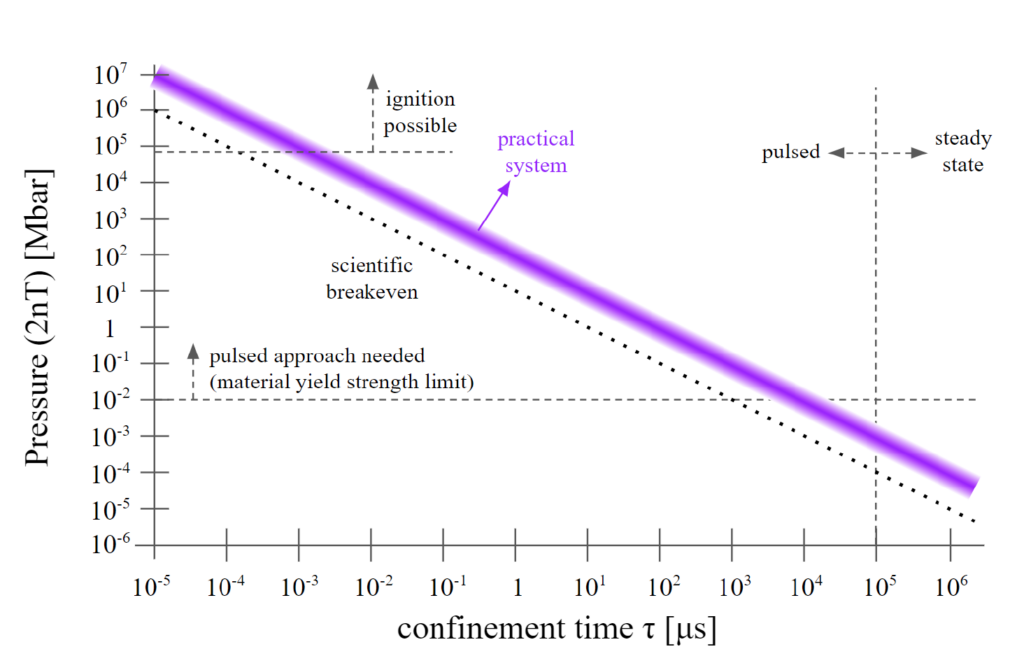
Recent Progress. In the past few years, there have been exciting developments that significantly raise the prospects that practical commercial fusion power can be achieved. Commercial activity has grown remarkably, with more than 30 startups worldwide, including a few with substantial funding (>$100 million). In addition, tools for designing and running fusion experiments have improved, notably recent advances in simulation and modeling tools that enable faster iteration and greater predictability.
Four approaches based on D-T fusion are currently well-supported in industry and/or government:
- steady-state magnetic confinement.
- short-pulse laser-driven inertial confinement.
- long-pulse mass confinement.
- long-pulse magnetic confinement.
Several companies are also pursuing approaches involving non-D-T fuel.
Some major recent milestones include:
- In September 2021, Commonwealth Fusion Systems showed how the high magnetic-field strength needed for compact steady-state magnetic confinement fusion could be efficiently achieved with high-temperature superconducting tape.
- In December 2022, the National Ignition Facility (NIF) at Lawrence Livermore National Laboratory provided the first demonstration of controlled ignition of fusion reactions.
Major challenges remain. Despite the exciting progress, there remain major scientific, engineering and economic challenges to creating working, inexpensive, scalable fusion energy systems that can address the world’s urgent energy needs. The challenges include:
- achieving overall facility gain (that is, more energy out than in at the system level)
- avoiding rapid loss of confinement
- resilience against gradual component degradation
- systems with a wide range of rated power capacity, from small (10 MW) to large (2 GW)
- scalability and mass production of systems
- low cost of energy produced
Given the enormous transformative potential of commercial fusion power, the world should be undertaking a broad range of critical-mass efforts—including continuing to advance existing efforts and identifying additional approaches.
Two attractive approaches. Science for America therefore systematically reviewed the possible approaches to fusion to identify approaches that are currently receiving relatively little attention, but which recent evidence suggests could provide promising paths to commercial fusion.
In this White Paper, we describe two such approaches that are supported by solid scientific evidence from recent experiments and have the potential for both simpler engineering and lower cost. These approaches involve:
- short-pulse magnetically-driven inertial confinement.
- intermediate-pulse magnetic confinement.
Figure B shows a Pτ plot displaying these two approaches relative to the four currently well-supported approaches.
Fig B: This figure shows four currently well-supported fusion approaches (steady-state magnetic, short-pulse laser, long-pulse mass, and long-pulse magnetic) as well as two promising approaches currently receiving little attention: short-pulse magnetic (Magnetic Igniter) and intermediate-pulse magnetic (Sweet-Spot Burner). As discussed later, estimated peak Pτ demonstrated for different approaches in these regimes is also shown.
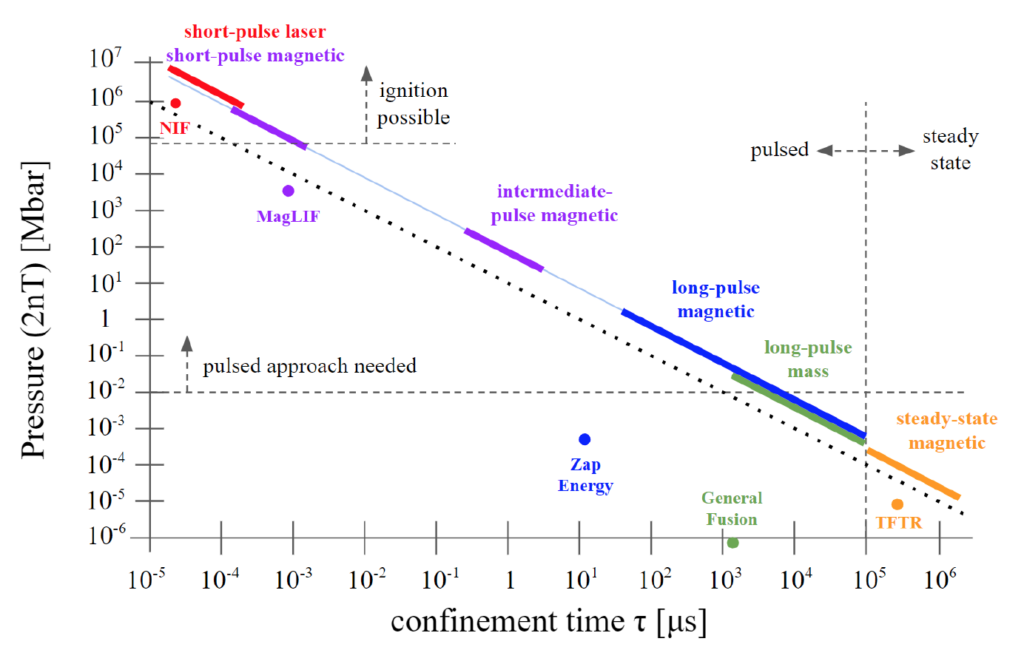
As a shorthand, we refer to a possible energy system using these approaches, respectively, as a “Magnetic Igniter” and a “Sweet-spot Burner.”
We briefly outline important features of each approach.
Magnetic Igniter. The concept is to use short-pulse magnetically-driven inertial confinement not just to achieve a burning fusion plasma, but to achieve ignition. Ignition is attractive because it is the point at which the system can burn additional unheated fuel, with the result that fairly small increases in energy input lead to huge increases in energy output.
The concept builds on learning from two recent experimental achievements: (i) The National Ignition Facility’s landmark demonstration in 2022 that ignition can be achieved by using laser-driven inertial confinement; and (ii) high-performance MagLIF experiments on Sandia’s Z Machine in 2022 using magnetically-driven inertial confinement to implode a cylinder of magnetized preheated fuel. Together, these two experiments demonstrated, respectively, the highest and second highest Pτ value ever achieved in the laboratory.
Our analysis indicates that short pulse magnetically-driven inertial confinement offers many potential advantages compared to laser-driven fusion. These advantages include the potential for much higher efficiency in energy delivery (>10x), much smaller scale (<1/10), much lower cost (<1/10), and simpler/cheaper targets. The minimum viable scale for the Magnetic Igniter may be in the range of ~100 MW, while also allowing much larger scales.
Sweet-Spot Burner. The concept is to use intermediate-pulse magnetic confinement (~1 μs pulses) to build a system with certain attractive properties. As described in the White Paper, the Sweet-Spot Burner design sits in the “sweet spot” that balances the minimum required energy and minimum required power for a fusion energy system, with the result that it could offer a very low minimum viable scale and cost.
For example, it should be feasible to create small energy systems (with power as low as ~10 MW, with eventual capital costs as low as tens of millions of dollars), as well as much larger systems (with power greater than or equal to 1 GW).
The possibility of small, compact, inexpensive energy systems would enable faster development cycles, expand the range of applications, broaden the customer base, allow application of mass production to drive down costs, and speed deployment around the world.
Single Demonstration Platform. Because both approaches involve pulsed magnetic fields, they involve many common components. In fact, they have enough similarities that it should be possible to demonstrate both approaches on a common pulser machine with programmable discharge timing.
With a concerted effort, it may be possible to: (i) efficiently build such a machine and demonstrate the ability to achieve net-positive facility gain and (ii) design and mass-manufacture inexpensive components with properties and costs suitable for use in commercial energy systems. (Commercial energy systems would be optimized separately for each approach and for various scales.)
Conclusion. Our examination of the fusion landscape has highlighted two promising approaches that have received comparatively little support. These two approaches — short-pulse magnetically-driven inertial confinement (Magnetic Igniter) and intermediate-pulse magnetic confinement (Sweet-Spot Burner) — are supported by recent compelling experimental evidence and offer potential engineering advantages that may facilitate development of viable commercial systems. We hope that the White Paper’s discussion of these concepts – their potential advantages, challenges, and questions about how to best investigate them – will stimulate research efforts in the fusion community that complement existing fusion research efforts and accelerate fusion power as an affordable, scalable clean energy source.
To read the full white paper, click here